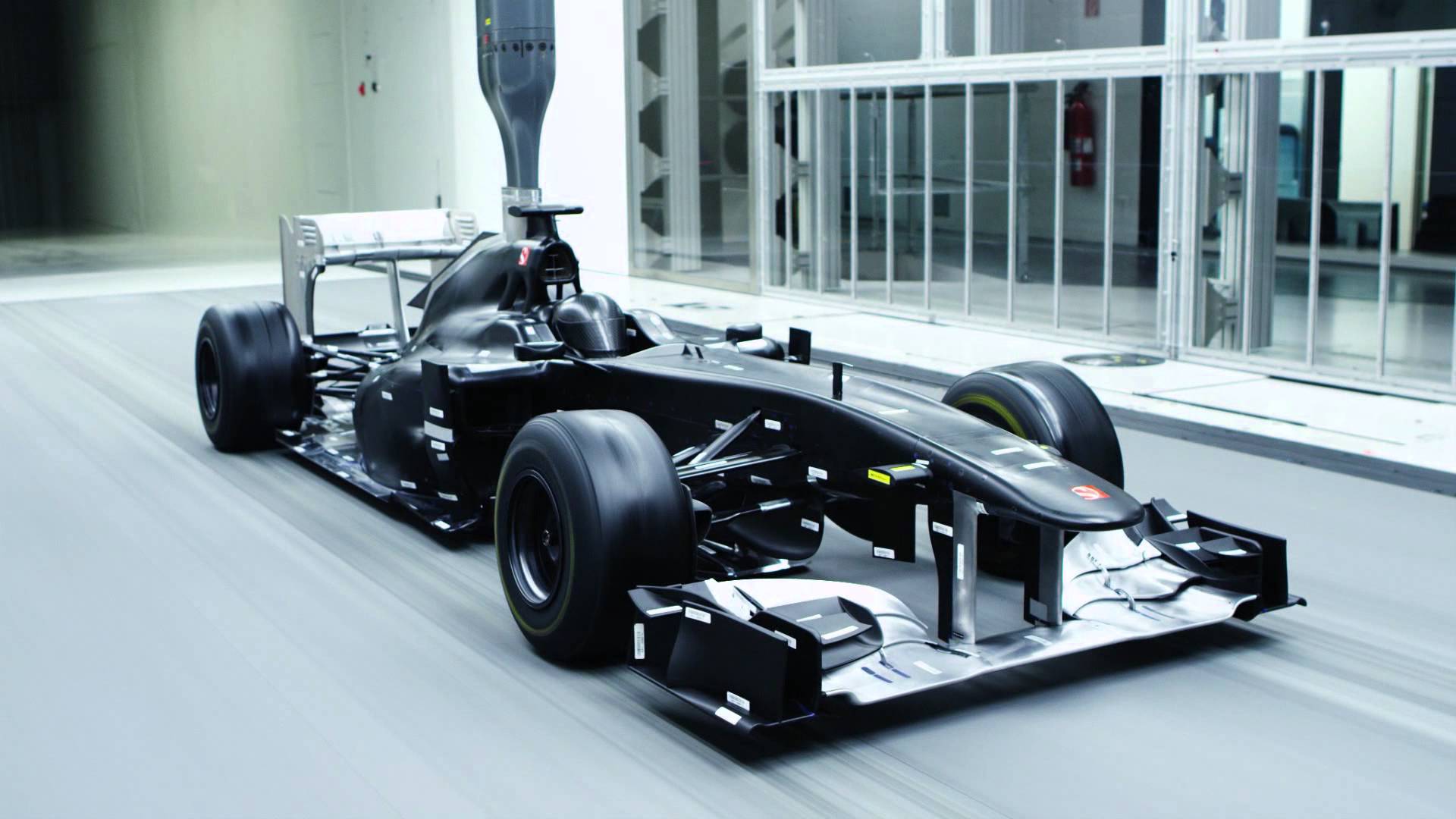
Race cars in general have a peculiar shape that distinguishes it from any road car on the planet. This shape might be different in each one of the wide range of motorsport categories around the world, but some aspects are still present, regardless of category. Have you ever wondered why?
If you look back at the dawning of road racing and compare the race cars of the time with the representatives of each decade from then to now, you’re gonna see a range of different shapes and parts, but a single aspect will be common to all of them: all the shapes show the concern with aerodynamics.
Aerodynamics is study of the forces and moments created by the interaction of air with a solid body, such as an airfoil. It is a branch of fluid dynamics and relevant studies on the area began in the eighteenth century, but observations of fundamental phenomenon have been recorded much earlier.
Most of the early efforts in understanding aerodynamics were directed to the development of heavier-than-air flight, which is the kind of flight that does not use buoyancy effects to generate lift forces. Since then, the use of mathematical analysis, wind tunnel tests and computational simulation have evolved in an astonishing manner, helping aerodynamics to become crucial in many other fields.
How Strong Can Air Be?
You might be wondering how important aerodynamic forces may be, since it is created only by air, which doesn’t even seem to have weight (although it has). In fact, you can feel how much aerodynamic impact is when you stick your hand outside of moving car’s window. If the car has enough speed, it might be rather difficult for you to keep your hand pointing forward.
Also you have to keep in mind that aircrafts, that can weight tenths of tons, are kept in the air solely by the interaction between air the plane’s wings and control surfaces. Furthermore, you probably have heard of the disastrous consequences of tornadoes and hurricanes, which in short, are caused by wind interactions with solid objects.
A practical example of how important aerodynamic forces might be in motorsport environment, can be observed by comparing the cross-section of suspension wishbones of open-wheel race cars in 70s and nowadays. The recent cars have streamlined, airfoil-shaped wishbones, while the cars in the 70s have rough circular cross-section. Figure 1 shows the comparison between a small cylinder and a 10 times larger airfoil. It might be hard to believe, but both these shapes have the same drag.

Figure 1. Round tube cross-section and much larger airfoil cross-section have the same drag. (Race Car Aerodynamics, Designing for Speed – Joseph Katz)
To help improve your understanding of aerodynamics, let us first discuss some force definitions. Figure 2 shows a racing car with the three aerodynamic forces that arise around it.

Figure 2. Aerodynamic forces on a race car. (Race Car Aerodynamics, Designing for Speed – Joseph Katz)
Drag is the resultant of aerodynamic forces that acts in the longitudinal axis of the car, opposing its movement. This is a crucial element of aerodynamics study, and it is of primary concern in road cars aerodynamic design. It must be overcome by the tractive force generated by the engine.
Lift is the resultant of aerodynamic forces that acts upward. Lift reduces the vertical forces of the car, and its reduction is of primary concern in racing car aerodynamics study. The opposite of lift is downforce, which is the resultant of aerodynamic forces that pushes the car against the ground.
Side Force is the resultant of aerodynamic forces that pushes the car sideways. This force is generated by lateral winds acting upon the vehicle. It is important for stability studies on road cars, but since lateral wind components are relatively small in racing vehicles, its importance is reduced in motorsport environment.
Why Are Race Teams That Concerned About Downforce?
Downforce generation has become one of the major performance-defining factors (along with tyre development) in the last 40 years or so. If you have read my previous article on lateral force generation by the tyres, the benefits of downforce will be clear: the amount of lateral force that can be generated by a tyre is extremely dependent on the vertical force acting upon it.
Simply put, the higher the vertical loads on a tyre, the higher the lateral force it can generate. One can think that the performance of a tyre can be improved by increasing the weight of the car, since it would augment the vertical load on the tyre, but this is not true.
As previously stated on the article about lateral weight transfer, the higher the weight of a car, the higher the weight transfer would be, and hence, the lateral force produced would be smaller. Also, a heavier car would require higher lateral forces to withstand the same lateral acceleration, and hence the benefit of higher vertical loads on the tyres is lost. Furthermore, a heavier car would have less straight-line acceleration, and the overall effect would be higher lap times.
Aerodynamic downforce on the other hand, increases vertical load on tyres without much penalties, except for the commonly added drag that comes with it. The reason why race teams are favouring downforce generation instead of drag reduction, is that, even though straight-line speeds are reduced, the speeds on corner increase so much, that the overall lap time is reduced.
Of course, the above is true depending on the race venue visited in the particular racing weekend, but it works for the major part of circuits. Notable exceptions are circuits where the straights or corners with large turn radius correspond to a much larger part of the circuit, like Monza, Spa-Francorchamps and Montreal tracks. This creates the need for variations of a car aerodynamic configuration along the season. Figures 3 and 4 shows the rear wing of Toro Rosso STR5, Toro Rosso’s F1 car for the 2010 season, during the Grands Prix of Italy and Bahrain, respectively.
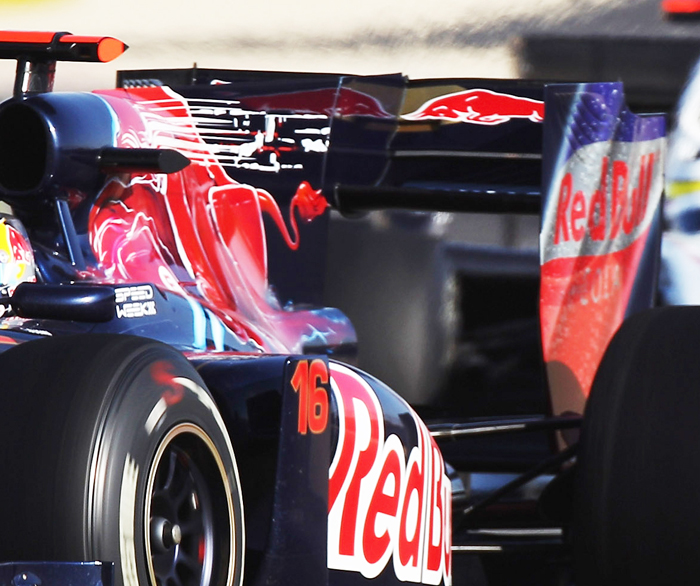
Figure 4. Toro Rosso STR5, Bahrain rear wing configuration.
(formula1techandart.files.wordpress.com)
How Aerodynamics Affect Vehicle Shape
In early forms of motor racing, drag reduction was the only aerodynamics-related concern. In the beginning of nineteenth century, racing cars used to have a streamlined, cigar-like shape, primarily to reduce drag. The beginning of motorsport saw great developments in other areas, like tyre and engine technology, so, it is difficult to quantify how much benefit of the cars performance came from drag reduction in circuit racing cars. It is known, though, that drag reduction was the major factor for the performance of land speed record-breaking cars.
With the ever growing concern to reduce aerodynamic drag, shapes increasingly streamlined started to arise. The entire car was design in order to provide a smooth, disturbance-free airflow around the car. This lead to generation of aerodynamic lift on race cars. This reduced the available grip. That might have been sensed by the drivers at the time as a loss of speed on corners and a light feeling on the steering wheel, but aerodynamicists would argue that only drag was an important parameter.
The first efforts of introducing downforce generation (or at least lift reduction) in motor racing are credited to Swiss engineer Michael May, in 1956. He realized that airplanes would keep themselves in the air through the generation of tons of lift through their wings, and that the principle could be applied to generate downforce by installing a wing upside down.
May then installed an inverted wing acting through the CG of his Porsche Spyder. The wing could be tilted from angle of attitude of -3° (nose up) to +17° (nose down), but the scrutineers deemed the concept illegal, and the first car with wings to provide downforce never raced.
Meanwhile, prototype sports cars would take benefit of their large plan areas and top speeds (as you will see in a later post, these are major factors contributing to the rise of aerodynamic forces) to generate downforce through the fitting of spoilers to disrupt smooth lift-generating airflow. This was the first time the racing world saw lap times reduction through lift-reducing/downforce-generating aerodynamics.
It was then, when American engineer Jim Hall remembered once again (most of the world had either forgotten or never heard of Michael May’s earlier attempts) that wings can keep heavy aircrafts in the air, and the concept could be adapted to generate downforce. Hall then created the Chaparral 2E CanAm race car, credited to be the first car to ever race with wings to generate downforce in 1966. It is said that the Chaparral 2E established the paradigm for virtually every other race car built ever since. Figure 5 shows the car.
It was the beginning of a revolution. Formula One quickly saw the benefits of installing inverted wings on racing cars, and simple fabrications of aluminium mounted on thin struts appeared, as shown in the first Formula 1 Car to ever race with wings, figure 6. The first wings on Formula One were really poor structurally designed, and that caused many accidents due to structural failure of either wings or wing supports, which lead CSI, Formula One regulating body at the time, to ban wings altogether.

Figure 6. Lotus 49B, winner of 1968 Formula One Season Drivers and Constructors Championships. (www.lotusespritturbo.com)
After some rethinking by CSI and F1 constructors, wings returned, but this time, with stricter regulations related to wings and wing supports structural construction, imposing safer shapes to arise.
It was when Jim Hall (yes, him again!) realized that the large plan areas of the prototype cars would allow him to generate a huge amount of downforce, if he could create a low pressure zone underneath the car. This was the beginning of ground effect aerodynamics on racing cars, as Hall created the Chaparral 2J, also known as the “sucker car”.
The concept was simple: Hall used two large fans powered by auxiliary engines to remove air from the underbody, while ‘side skirts’ (as they got know) would seal the lateral of the car, avoiding the circulation of airflow under the car and creating a low pressure zone underneath it. This low pressure combined with the large plan area of the car created massive amounts of downforce, hitherto unknown. Chaparral 2J is shown in figure 7.
The concept was once again copied to Formula One, as Peter Wright and Colin Chapman created the Lotus 78. The car did not use fans but instead, the whole underbody of the car was shaped as a wing. The key principle here, is that a wing in close proximity to the ground will generate higher lift, but the concept also applies to an inverted wing generating downforce. Figure 8 illustrates.
The other teams tried to play catch up, but the best answer to Lotus 78 was introduced by Gordon Murray in his Brabham BT46B, largely known as the ‘fan car’. At the time, Formula One regulations forbade devices whose primary function was aerodynamic to move relative to the car (this rule was introduced in order to avoid earlier attempts of changing angle of incidence, to reduce drag on straights). However, Murray installed a fan that removed air from the underbody, just as in the Chaparral 2J, and alleged that the primary function of the fan was to cool the engine, which allowed the car to race once and win, before being banned. Brabham BT46B is shown in figure 9.
The first attempt to ban ground effect from Formula One was made in 1983 with the introduction of mandatory flat bottoms. At the time, the cars were running 1.5 litre turbocharged engines that could generate four figures power levels. With strong need for downforce generation in order to improve traction so that the tyres could cope with the power from the engines, and low concern to drag, this era saw the increasing use of larger wings. But in 1989, turbo engines were also banned, and teams had to once again look for ways of increasing downforce without much drag penalty.
Then engineers found out that by using extremely low ground clearances, and with enough nose-down attitude, massive amounts of downforce could be generated: ground effect was back. Later, diffusers came into play, to fill the need of improving airflow exit from the underbody.
In the first years of the 90s, Formula One saw the use of extremely low ride heights and astonishing stiffer suspension springs, in order to control ground clearance. The demand for ride height control caused the springs to be so stiffer that the cars would be almost no sprung at all, relying only on tyres to cushion any roughness from the road!
The concept was improved with the introduction of active suspension by Williams, in 1992 season, with the FW14B. The concept was to use electronically-controlled hydraulic actuators to control ride height, pitch and roll attitudes of the car. The primary purpose of active suspension was actually aerodynamic, rendering it illegal, but it was alleged that the primary role of the system was to improve handling. The video below shows the effect on the Williams FW15C, the 1993 successor.
Williams FW15C
In 1994, active suspensions were banned in F1, but the development of underbody aerodynamics went on until 1995, when the introduction of stepped floor by FIA, the F1 regulating body from the time to present, substantially reduced the downforce generated by ground effects. This made the cars less sensitive to attitude and ride height, making them safer and more predictable to drive.
Contemporary Formula Racing saw the increased difficulty for overtaking to occur. There are some beliefs that this has been caused by aerodynamics, and drivers sometimes complain that once they get close behind another car in a corner, in an attempt to overtake by drafting on the next straight, they lose so much grip due the wake of the car ahead, that it becomes impossible to keep following the car closely. Also, some believe that the reduction in braking distances caused by the rise in downforce also makes overtaking under brakes much more difficult.
With that in mind, FIA commanded studies on aerodynamics of cars following each other closely, in an attempt to find a set of rules that would allow close running and overtaking to occur. The studies seemed to indicate that if total downforce was reduced, instead of facilitating cars to follow each other, it would make things harder for the trailing driver.
In 2007, FIA setup the so called “Overtaking Working Group” (OWG) in attempt to understand why it has become so difficult to F1 cars to run closely to each other. The group was composed by the then McLaren engineering directors Paddy-Lowe and Renault F1’s Pat Symonds and was headed by Ferrari consultant Rory Byrne, assisted by aerodynamicist Jean-Claude Migeot. The group focused on increasing mechanical grip by the tyres while reducing the role of aerodynamics, resulting in 2009 regulations that made F1 cars look rather different.
On the next posts about aerodynamics, I will talk more about how aerodynamic forces are created and some tricks the engineers use to increase downforce, along with some discussion on recent relevant aerodynamic innovations. Keep in touch with us! Subscribe to our email list below, so that you will never miss an article here! 🙂 See ya!
KEEP UP TO DATE